Geodynamics of the Earth
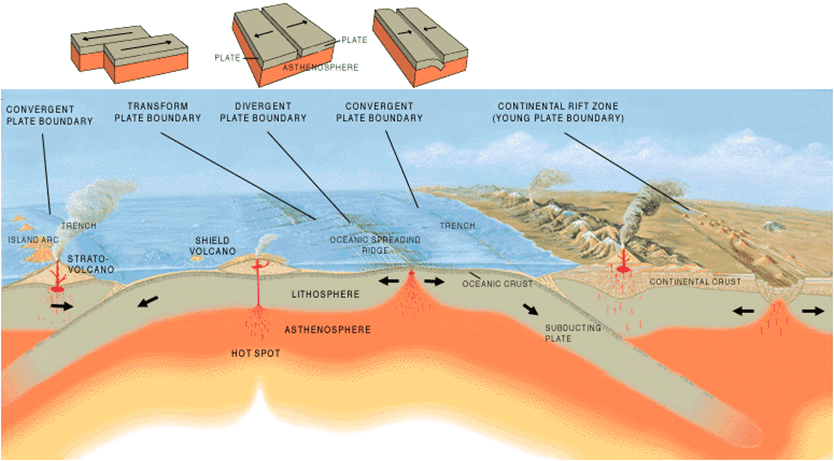
Basics of building the earth's body and Geodynamics
Physical characteristics of the Earth
The physical properties of the Earth’s body are measurable, allowing us to determine its size, shape, mass, gravitational and magnetic fields, geomagnetic flux, and more with great precision. The Earth’s interior, however, can only be examined indirectly using seismic waves (earthquakes), which penetrate the Earth’s structure similarly to X-rays.
**Surface Features of the Earth according to Source1:**
**Continents** – These consist of landmasses, the continental shelf (with a slope of approximately 0°07′ and a depth ranging from 20 to 550 m), and the submerged continental slope (with a slope of approximately 5°). About one-quarter of the continental area is covered by water. The following structural units can be observed on continents:
1. Precambrian shields
2. Platforms
3. Folded mountain ranges extending along orogenic belts in deformed rocks (see the following image).
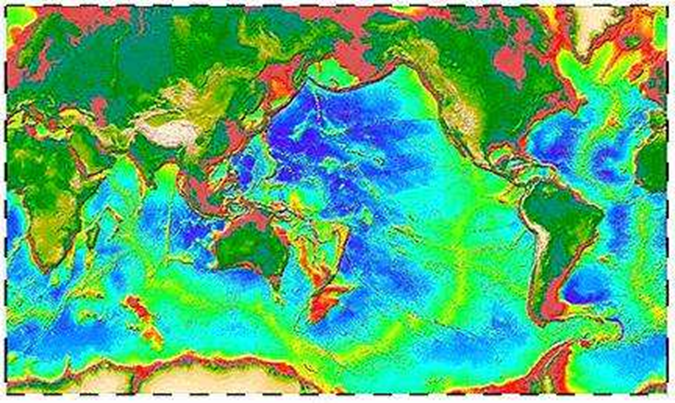
**Continental Margin** – This is the transition zone between the continent and the ocean basin. It includes:
– **Atlantic-type margin** – Consists of the continental shelf, slope, and rise.
– **Andean-type margin** – Features a narrow continental shelf with an adjacent oceanic trench directly beneath the continental slope.
– **Island arc-type margin** – Composed of a volcanic island arc and an associated oceanic trench.
**Oceans** – Deep-sea basins generally have depths of about 6 km, with trenches reaching 9 km and, in rare cases, up to 11 km. The ocean’s equivalent of continental mountain ranges is the **mid-ocean ridges**, rising about 3 km above the ocean floor. A central **rift valley** often runs along the middle of these ridges. Elevated areas compared to deep-sea **basins** are known as **continental rises**. Oceans also contain the previously mentioned **trenches**, adjacent **island arcs**, **volcanoes**, and **volcanic ridges** (see illustration).
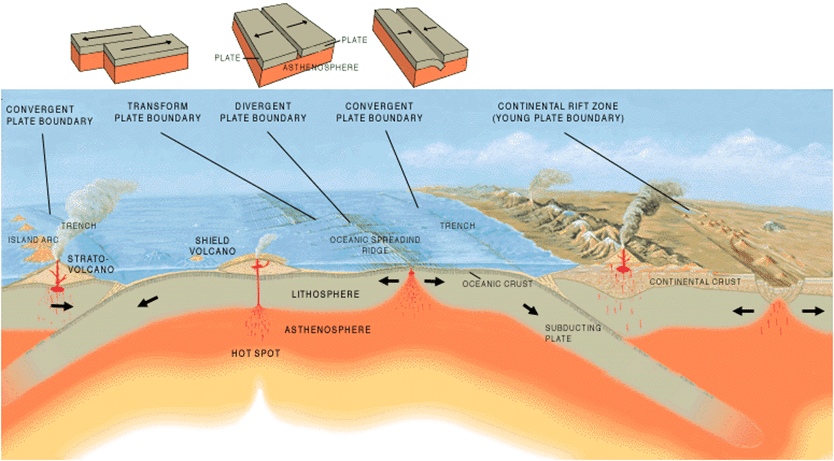
### **Volcanic Distribution**
75% of currently or historically active volcanoes are concentrated along the circum-Pacific belt, also known as the **”Ring of Fire.”** This belt includes the young mountain ranges of western America and volcanic island arcs bordering the northern and western Pacific. The second major belt is the **Mediterranean-Asian belt**, where volcanic activity is much less frequent, except in **Indonesia** and the **Mediterranean**. Oceanic volcanic belts were mentioned earlier.
It is important to note that volcanic activity is also linked to **rift systems** (both oceanic and continental). Studies have shown that 93% of all active volcanoes are located along circles formed by three mutually perpendicular planes passing through the Earth’s center.
### **Seismic Activity Distribution**
1. **Shallow earthquakes** – Focus depth up to **70 km**.
2. **Intermediate-depth earthquakes** – Focus depth between **70–300 km**.
3. **Deep earthquakes** – Focus depth between **300–700 km**.
The **circum-Pacific belt** contains **80% of all shallow earthquakes, 90% of intermediate-depth earthquakes, and nearly all deep earthquakes.** The remaining seismic activity occurs in the **Mediterranean-Asian belt** and the **global rift system**.
Seismology and the Earth's interior
Seismology studies earthquakes, both natural ones caused by Earth’s geodynamic phenomena and those artificially induced by human activities. We briefly covered seismology in the previous article Geophysics (kint.cz).
Seismic waves travel through the Earth’s body and return to the surface carrying information about the physical properties of Earth’s interior. A disadvantage of natural earthquakes is that we do not know either the exact time or location of their occurrence. This disadvantage does not apply to underground nuclear explosions 4.
Since the speed of seismic waves depends, among other factors, on density, basic considerations about density distribution within Earth can be made. The Earth is density-inhomogeneous, with densities in its interior exceeding 5.5 gcm-3. Additional information on the distribution of mass inside the Earth is provided by its moment of inertia.
It has been determined that the moment of inertia of the Earth is 0.331 MR2 (where M is mass and R is the Earth’s radius), which implies that Earth’s mass is more concentrated around its center and that densities at Earth’s core are higher than average.
Seismic rays must therefore travel through the Earth along curved paths, reflecting off various seismic interfaces. The energy released during an earthquake or nuclear explosion is converted into different types of seismic waves. Longitudinal P-waves travel at twice the speed of transverse S-waves. On Earth, surface waves L are also significant, and they come in two types.
Rayleigh surface waves are similar to longitudinal waves, whereas Love waves correspond to transverse waves. The energy of long-wavelength waves penetrates deeper and propagates faster than that of short-wavelength waves 5.
The propagation of seismic rays through Earth is illustrated in the next image, which also shows Bullen’s model of Earth, consisting of three main parts: the upper mantle, mantle, and core; each part is further subdivided. Today, the division of the mantle into the lithosphere and asthenosphere is more commonly used. The upper part of the lithosphere forms the Earth’s crust 6.
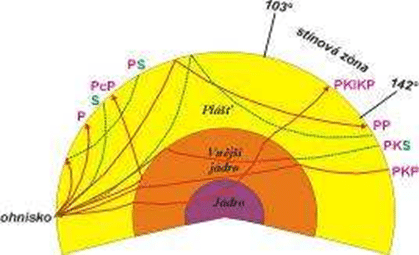
Seismic rays, originating from the focus, curve as they go deeper. S rays are more curved than P rays. S rays end—they do not pass through the core, but instead reflect off it. The core behaves like a liquid, which is reflected in the fact that it does not allow S waves to pass through. 7.
The most prominent boundary is the outer limit of the core. The Earth’s core was discovered by Gutenberg through the observation of the “seismic shadow.” For example, if the epicenter of an earthquake were to lie to the west, P waves could be registered across the entire globe except for a belt on the southern hemisphere between southern latitude 103° and 142°, where P waves are very weak.
This is because the P ray, which tangentially passes around the core, hits beyond 142°, while the immediately neighboring ray breaks into the core and exits to the surface in the shadow zone. 8.
By studying the arrival times of seismic waves, especially from nuclear explosions, at various distances from the epicenter, hodographs could be constructed, and from their slopes, the dependence of seismic wave speeds on depth could be determined. In places where the speeds change abruptly, the boundary of the Bullen Earth model lies. 9 (see figure).
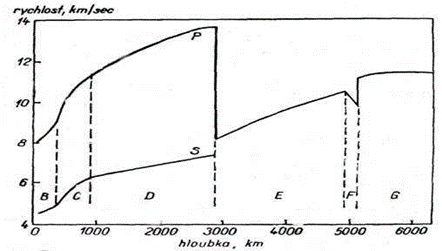
Bullen’s model of the Earth was also used for calculating other physical parameters: pressure, temperature (from 2,000 to 6,500 °C at the Earth’s center).
From the perspective of the Earth’s structural development, the most significant is the lithosphere (i.e., the Earth’s crust and upper mantle). The boundary between them is the Mohorovičić discontinuity (MOHO), where the seismic wave velocity increases abruptly.
Earth’s crust – it has very different thickness and composition. Under the continents, it consists of a granite and basalt layer; the boundary between them is the Conrad discontinuity, whose significance and proof are ambiguous. The average thickness of the crust is 35 km.
Under the oceans, there is only a basalt layer, and the average thickness of the crust is only 5 km. MOHO is the boundary where the chemical composition of rocks or their phase state, density, and seismic wave velocities change. 11.
The upper mantle is not homogeneous in the horizontal direction (i.e., the direction of P wave velocities n). However, even more significant changes occur in the vertical direction, where there exists a low-velocity layer. According to some authors, there are even 4 low-velocity layers down to a depth of 700 km. 12.
Based on research and according to the above source, the low-velocity layer can be characterized as follows:
- It lies at a depth of 60 – 250 km, and its boundary is not sharp.
- It extends around the entire Earth.
- Seismic waves are more slowed down under the oceans than under the continents. The low-velocity layer lies closer to the Earth’s surface under the oceans than under the continents. This provides further evidence that differences between oceanic and continental areas do not only limit themselves to the Earth’s crust up to MOHO.
- S waves of shorter wavelengths are absorbed. This means that rocks in the low-velocity layer are in a nearly plastic state, which likely indicates temperatures close to the melting point.
- Volcanoes are mostly found in places where earthquakes occur. Earthquakes associated with volcanism typically originate from depths of 60 – 200 km. This suggests that volcanic activity is linked to faults in the low-velocity layer, which is likely the source of primary basaltic magma.
- However, most earthquakes occur to a depth of 60 km, or at greater depths, the material becomes more plastic, allowing anomalous stress to be largely balanced by the “creeping” of the material.
The Earth’s crust and part of the upper mantle down to the low-velocity layer are collectively called the lithosphere (lithos – Greek for stone), consisting of rigid rocks. Beneath the lithosphere lies the asthenosphere (asthenos – Greek for soft); it is characterized by plastic rocks; it includes the entire low-velocity layer; its lower boundary is quite variable according to different authors.
The low-velocity layer plays a crucial role in the interpretation of isostasy, tectonics, orogeny, continental drift, and the tectonics of lithospheric plates (plate tectonics).
Physical properties of the Earth
Geodynamics deals with other physical properties of the Earth, particularly the following: density distribution inside the Earth; elastic properties such as pressure and gravitational acceleration (which can be derived from seismic wave velocities and the density distribution inside the Earth); temperatures within the Earth.
Earth temperatures
The current thermal state of the Earth results from its thermal history, about which a number of hypotheses have been proposed. These hypotheses are based on certain assumptions about the origin and early history of the Earth, its chemical composition, and the physical properties of the material from which the Earth is composed. 13.
The relationship between depth and temperature in the Earth is expressed by geotherm. The sources of internal heat are assumed to be:
gravitational energy, which was converted into heat during the formation of the Earth’s core,
energy generated by friction during tectonic subductions or slumps, which is transformed into thermal energy, likely in weakened zones such as the low-velocity layer or subduction zones,
thermal energy generated by the decay of radioactive elements. This is probably the main source that maintains the Earth’s external thermal state and the current heat flow:
– heat flow from the Earth’s interior (conduction, radiation, convection); its deep measurements,
– deep temperature relationships (thermal conductivity k(z), distribution of heat sources A(z)),
– surface temperatures of the Earth (see images),
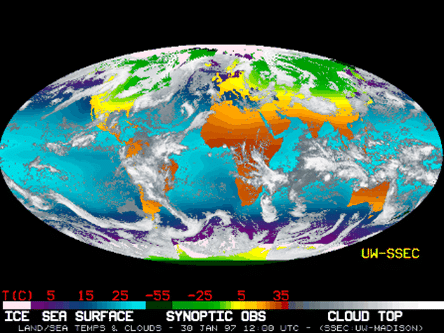
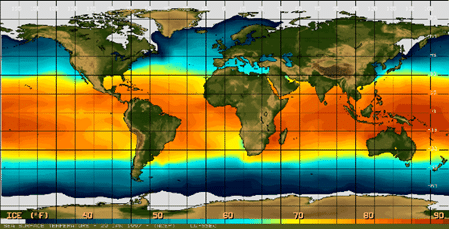
– viscosity of the individual layers of the Earth is known only approximately; what is particularly interesting to us is that many observations suggest that there exists a low-viscosity layer in the upper mantle at depths corresponding to the low-velocity layer 14,
– the Earth’s magnetic field – the lines of force of the magnetic field have a vertical direction at the magnetic poles, whose position changes over time (currently in northern Canada and Antarctica, their connection does not pass through the center of the Earth);
the cause of the field is 94% from within the Earth, with the remainder from external sources; the field can be expressed as the effect of several magnetic dipoles at the Earth’s center, having various orientations;
the first of these dipoles represents the main part of the regular Earth’s field, it is called the tilted geocentric dipole, whose axis forms an angle of 11.5° with the Earth’s rotation axis and intersects the Earth at the geomagnetic poles;
short-term periodic variations in the magnetic field (on the order of hours, days, or years) can be explained by electrical currents in the mantle and ionosphere; changes in intensity range from about 10 to 100 nT,
– magnetic storms – are short-term, irregular fluctuations, which may exceed the value of 103 nT; they occur in the ionosphere due to anomalous bombardment by solar radiation, mainly during the occurrence of sunspots 15, see figure,
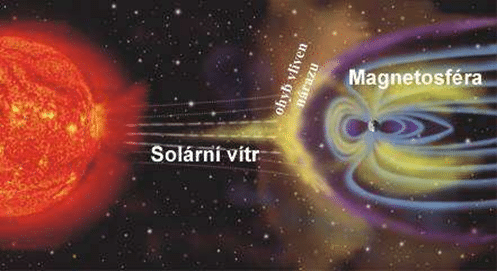
– secular changes manifest in declination, inclination, and intensity. Secular variations in intensity have values of units to tens of nT per year (iso-curve maps); cyclic changes in the direction of the field have a period of several hundred years and cause deviations of 10 to 20° on both sides from the average direction,
– Earth’s magnetic moment changes secularly; over 100 years it has decreased by 5%; however, it seems that the size of the moment oscillates around a certain value,
– paleomagnetism studies fossil and remanent magnetism; the movement of poles, magnetic inversions correlated with linear magnetic anomalies, are among the most important evidence for continental drift,
– Earth’s gravitational field – the mass of the Earth causes gravitational acceleration, rotation causes centrifugal acceleration; the vector sum of both accelerations is referred to as gravitational acceleration or weight; Earth’s rotation has caused it to be flattened at the poles; gravitational acceleration is a function of latitude – it is greatest at the poles and smallest at the equator;
– gravitational anomalies – from free air (excesses or deficits of mass inside the Earth compared to the normal distribution of mass, which corresponds to normal gravity);
Complete Bouguer anomalies express the effect of masses with anomalous density (i.e., density different from the average);
these masses are primarily located in the upper part of the lithosphere – it has been found that complete Bouguer anomalies are negative in high areas, whereas in oceanic regions, they are positive;
isostatic anomalies – zero anomaly indicates that the block is in hydrostatic equilibrium, a negative anomaly indicates that the block has excessively deep roots (Airy) or a too low average density (the block is hydrostatically pushed upward, and if no larger forces act in the opposite direction, the block rises), positive isostatic anomalies, on the other hand, indicate a tendency to sink.
Geological cycle and age of rocks
There are two sources of energy that drive geological processes: Earth’s internal heat and solar radiation. The internal source provides energy for the formation of igneous rocks, metamorphic and tectonic processes. The external source (the Sun) drives atmospheric and hydrospheric circulation, ventilation, erosion, and the formation of sedimentary rocks. Geochemical processes also play an important role.
The mutual relationship of all processes is expressed by the geological cycle (Figure 32), which is not closed. Magma, which forms in the mantle at depths of around 50 km and more, rises to the surface, enriching itself with additional materials containing silicates, allowing the formation of new rocks in the Earth’s crust.
At the same time, water and other gaseous components are released. From this, it can be inferred that the Earth’s crust, hydrosphere, and atmosphere formed gradually as a result of repeated magmatic activity.16
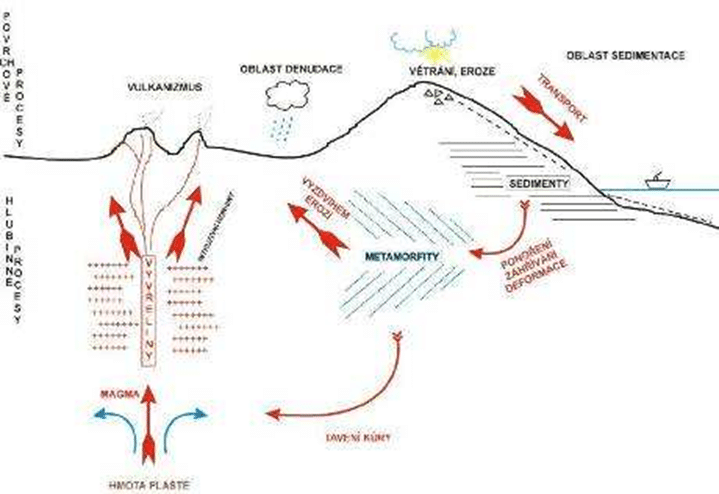
The age of rocks is determined either by paleontological methods based on fossils or by radioactive methods, and more recently also using paleomagnetism.
Lithosphere
The rheological behavior of the lithosphere and asthenosphere is described by viscosity and the law of decrease in deformation over time. A liquid heated from within or from below performs convective motion if the Rayleigh number exceeds the critical threshold.
The Rayleigh number for the mantle is significantly higher than the critical threshold for initiating convection. For thermal convection with a very high Rayleigh number, a thin thermal layer, the lithosphere, is characteristic.
From this perspective, the lithosphere is a thermal diffusive layer in the mantle convection system. This means that it is not very stable, and temperature distribution is governed by heat conduction, while in the mantle, temperatures are close to adiabatic temperatures. 17
In the lithosphere, we primarily observe its vertical and horizontal movements, sea-floor spreading, mid-ocean ridges and rifts, convection and rotation, convection in the mantle, and gravitational field (tectonic activity), the Mohorovičić discontinuity (Moho), Earth’s crust, the boundary between the lithosphere and asthenosphere. These areas are further described in the work 18.
Continental drift
Paleomagnetism provides data on drift. Properly selected samples of rocks of various ages show that the direction of their remanent magnetization is different from what it would be if they were magnetized in today’s Earth’s magnetic field.
From the direction of the remanent magnetization vector, the direction to the geomagnetic pole can also be determined. Based on these data, the positions of the paleopoles for individual geological periods can be reconstructed in relation to today’s geographic grid. The shift of the poles can be caused by:
1. The movement of parts or the entire Earth’s crust in a fixed position of the magnetic pole (or a fixed axis of Earth’s rotation).
2. The shifting of the magnetic pole in relation to the fixed position of the Earth’s crust.
Currently, paleomagnetic measurement results are often displayed by plotting paleomagnetic poles on today’s graphical grid with continents, connecting them with a smooth line to show how their position has changed. 19
The figure shows the paleomagnetic vectors of Cretaceous to Miocene rocks in the Carpathian region.
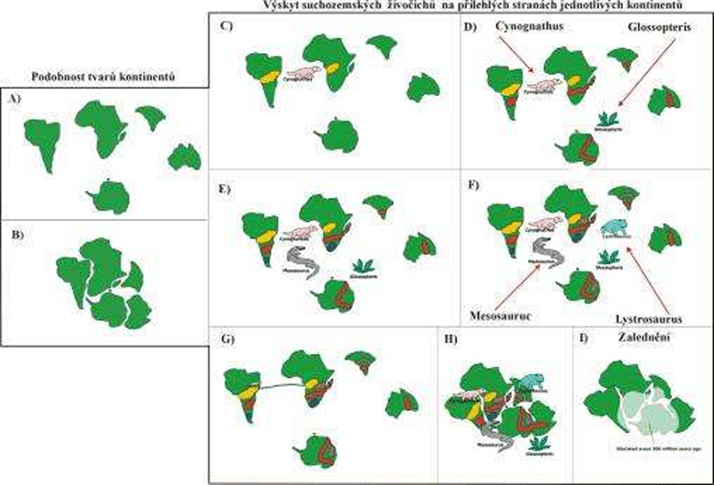
Lithospheric plate tectonics
The theory of continental drift and seafloor spreading were the cornerstones for the concept of plate tectonics, which is the new global tectonics.
This theory assumes that the surface part of the Earth consists of several rigid blocks that are in relative motion to each other (see figure). The blocks extend to a depth of about 100 km and slide over the asthenosphere. 20
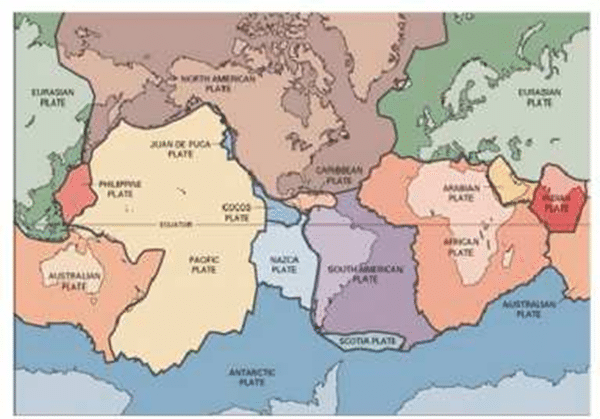
The boundaries of lithospheric plates were determined based on zones of increased seismicity. There are three basic types of boundaries: a) Extensive (divergent) boundaries, where the normal components of the forces acting on the plates move away from the boundary; b) Compressive (convergent) boundaries – the normal components of the forces point toward the boundary. Along extensive boundaries, new oceanic lithosphere is symmetrically created on both sides, while along convergent boundaries, the lithosphere asymmetrically sinks into the mantle (subduction).
This type of boundary is often accompanied by oceanic trenches, the formation of island arcs, and tectonic activity at the continental margins (e.g., the Alpine-Himalayan mountain range). c) The third type of boundary is transform faults. Their discovery is one of the important results of magnetic anomaly research in the northeastern part of the Pacific Ocean. The interpretation was provided by J. T. Wilson; he defined 10 types of transform faults. 21